Quantum Computing: Triple Qubit Entanglement Achieved in Research Breakthrough
Another step on the road towards quantum scalability
Researchers with the Riken Center for Emergent Matter Science in Japan have demonstrated a triple-qubit, silicon-based quantum computing mechanism - opening up the road for increased scalability beyond a mere increment in total qubits on a given system. Previously, qubits had only been shown working in entangled pairs -- and this research demonstrates that entanglement (and thus computation) can actually be divided between three qubits.
Quantum computing rests atop qubits - the quantum equivalent of the modern transistor. But while typical transistors can only represent one value at any point in time (with that value being either zero or one), qubits benefit from the superposition mechanic of quantum physics, meaning that they can represent both states at the same time.
Until now, quantum computing systems worked by entangling two distinct qubits, which allowed them work in tandem in solving any complex workload (entanglement meaning that the qubits perfectly mirror each other, and any changes to one qubit's state are instantly replicated in the other). If you think of each qubit as a single core, the research now increases the maximum amount of qubits ("cores") that can work in synchrony to be three, from its previous two-qubit maximum. Theoretically, you can now build multiple triple-core quantum computing subdivisions, instead of dual-core ones.
This research thus has several implications in quantum scaling, as well as in the complexity of quantum algorithms. Seigo Tarucha, one of the researchers involved, explains that "(...) two-qubit operation is good enough to perform fundamental logical calculations, but a three-qubit system is the minimum unit for scaling up and implementing error correction."
The researchers used one of the approaches currently being explored as enablers for quantum computing: a trio of silicon quantum dots, which are built out of a silicon/silicon–germanium heterostructure and are controlled through aluminum gates. Each silicon quantum dot features a single electron (negatively charged particles) which changes its spin states in response to a strong, on-chip magnet. The magnet generates a magnetic-field gradient which in turn separates the resonance frequencies of the three qubits, allowing them to be individually addressed.
This has important implications for error correcting of results. Turing machines like our personal computers already have deep error correction protocols inside them, which ensure the validity of calculations. This error correction requirement is still in its infancy in the quantum field, which is why this demonstration is so important. As per the researchers' approach, the third qubit can now be used as an aide in calculations, helping achieve a remarkably high (for quantum computing) state fidelity of 88%.
As with everything relating quantum computing, which is still in its nascent phase, scalability is the keyword here, and the researchers will continue to explore what they theorize can already be achieved with their technique.
Stay On the Cutting Edge: Get the Tom's Hardware Newsletter
Get Tom's Hardware's best news and in-depth reviews, straight to your inbox.
"“We plan to demonstrate primitive error correction using the three-qubit device and to fabricate devices with ten or more qubits," said Tarucha. "We then plan to develop 50 to 100 qubits and implement more sophisticated error-correction protocols, paving the way to a large-scale quantum computer within a decade.” The world awaits.
Francisco Pires is a freelance news writer for Tom's Hardware with a soft side for quantum computing.
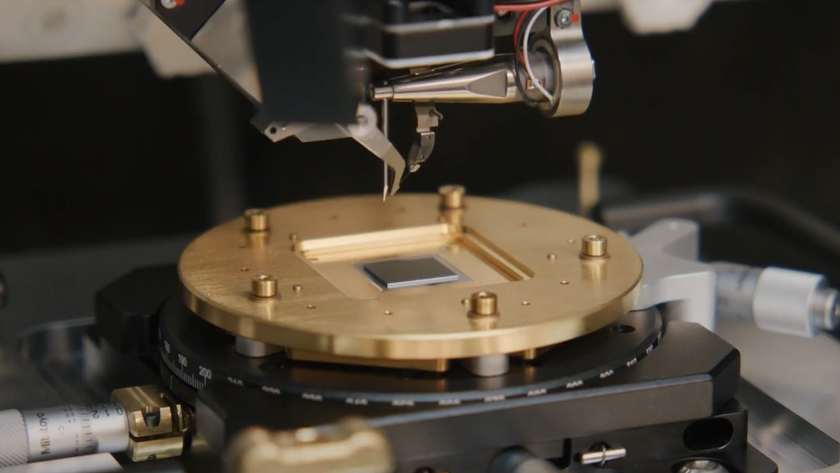
Amazon's Ocelot quantum chip uses 'cat qubits' to 'reduce error correction by up to 90%'
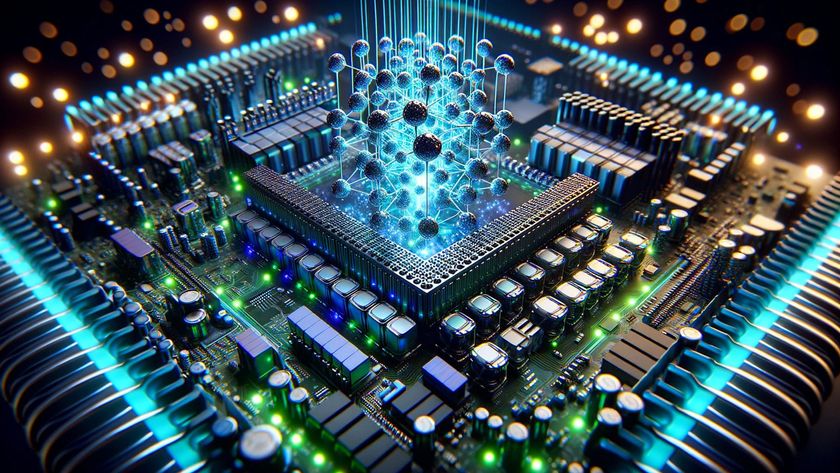
Quantum computing stocks tank as Nvidia CEO Jensen Huang predicts the tech won't be viable for another 20 years — stocks fell more than 40% for a total market value loss of over $8 billion
-
Grahaman27 I find it really interesting the author uses the term of "cores" for the number of states a qubit has. Is it just me or does this reek of incompetence on the topic?Reply